Deep dive🧜‍♀️: Carbon dioxide removal solutions
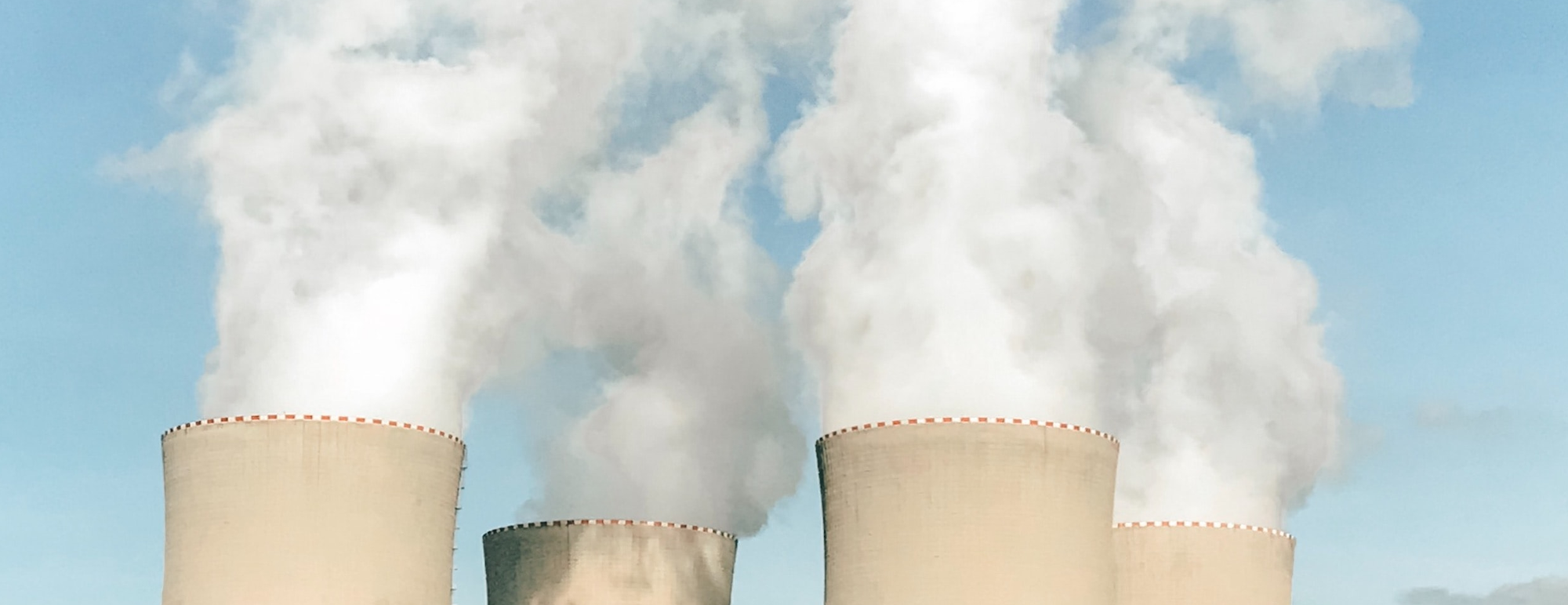
Carbon dioxide removal (CDR) solutions represent an emerging area of focus and innovation within the voluntary carbon market.
Indeed, the scaling of these solutions is critical to meeting the net zero aims of the Paris Agreement, with the latest IPCC report outlining a deployment rate of 5-16 giga-tonnes a year of by 2050.
This deep dive provides  a brief overview of seven of the most promising emerging carbon dioxide removal solutions:
- 🌳 1. Forestation
- 🌱 2. Soil carbon sequestration
- 🌊 3. Coastal blue carbon
- 🦑 4. Ocean based
- 🔥 5. Biochar
- đź—» 6. CO2 mineralization
- 🌀 7. Direct air capture and storage
Each is introduced and discussed in relation to their removal capacity, current cost and any barriers to implementation. This is followed by innovative examples of emerging projects and a case study of successful or scaled implementation.
This content originally featured in the deCarbonized newsletter from 22/01- 05/03/22.
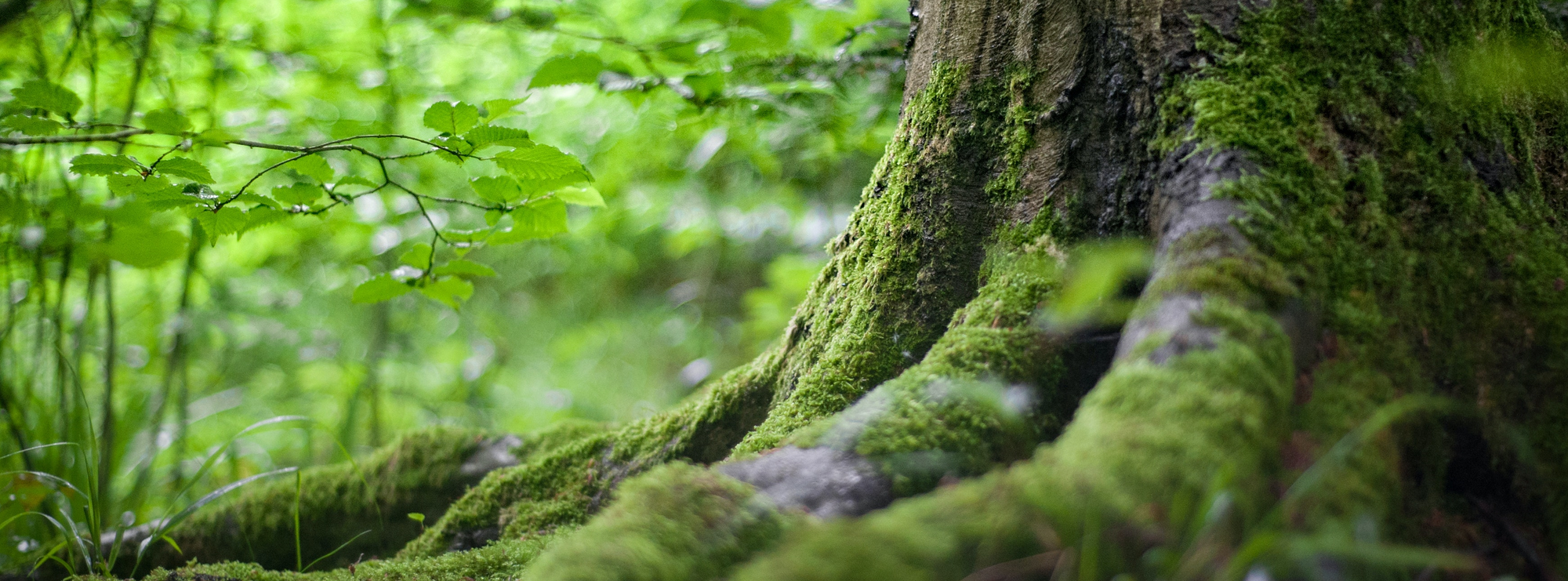
1. Forestation
Overview
Forests are responsible for removing nearly 30% of all anthropogenic CO2 emissions annually. They therefore hold great potential to drive purposeful, accelerated carbon dioxide removal (CDR).
It is important to highlight that stopping deforestation is more effective than a reforestation project. This is because existing ecosystems have reached greater maturity and complexity, and so able to sequester larger amounts of carbon while maintaining important ecosystem services, such as biodiversity and watershed management.
There are four main types of forestation project used in carbon offsetting:
- Restoration– the replanting of trees on recently deforested land to drive regeneration.
- Afforestation – the establishment of new trees on land where forests have not existed recently, often as monoculture plantations. Some ecologists question the feasibility and utility of these efforts.
- Agroforestry – practices that integrate trees into agricultural systems, alongside crops, livestock, or both.
- Improved forest management – active modification of forestry practices to promote greater forest biomass and carbon storage, i.e. improved tree plantation management.
The risks associated with forestation projects are higher than with other CDR solutions, which remove carbon into more stable biological or geological stores.
The risk of carbon leakage is particularly high, as any land preserved to store carbon is likely to lead to greater pressure to convert land surrounding areas.
Additionally, it can take up to 100 years for carbon sequestration potential to reach its peak. This requires long term and rigorous measuring, reporting, and validation (MRV) of forestation efforts to ensure this risk is appropriately managed.
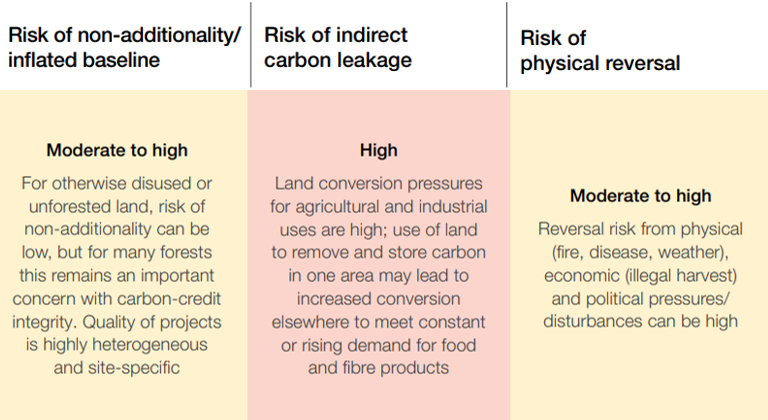
Despite these risks however, forestation projects drive sustainability benefits beyond carbon removal, actively contributing to the UN Sustainable Development Goals.
Co-benefits include:
- Improved biodiversity and species conservation
- Preserving ecosystem goods and services, like water purification and pollination
- Creating new resources and livelihood opportunities for local and indigenous communities
Potential removal capacity and cost
Globally, the potential for restoration, afforestation and forest management projects has been estimated at 4 -12 giga-tonnes per year.
2015- 2016 (the most recent years for which global data is available), existing projects removed 90 million tonnes of CO2 equivalent per year. Space Intelligence have developed an excellent map detailing the location of these projects, detailed below. The green dots represent tree planting and restoration projects, the purple dots forest protection projects.
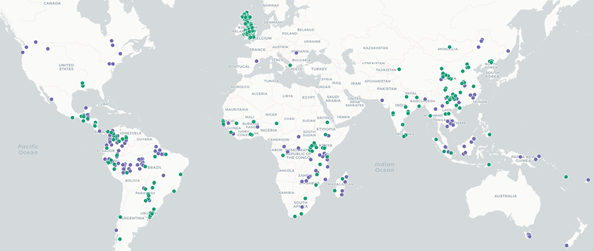
The maximum cost of afforestation and reforestation projects has been estimated at $100 a tonne. There is less agreement on the minimum cost, with estimates ranging from $1 to $20 a tonne.
Crucially, most estimates indicate that it is more costly to restore forests than to preserve existing ones, emphasizing the critical role of reducing deforestation compared to planting new forests.
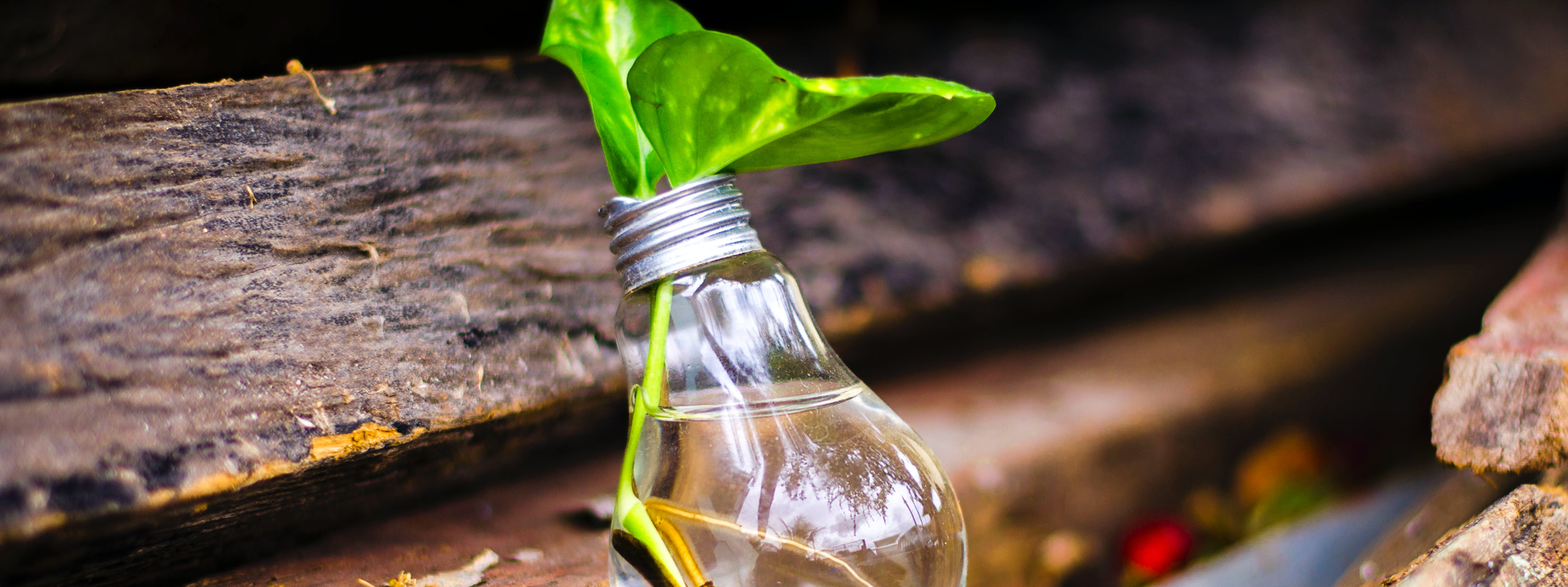
Innovative examples
- 774 smallholder families in Tanzania are being trained in sustainable agroforestry techniques, using carbon credit payments to offset costs and build climate resilience.
- In Oaxaca, Mexico, Rainforest Alliance certified coffee producers are seeking to quantify the climate benefits of sustainable plantation management to obtain carbon credits.
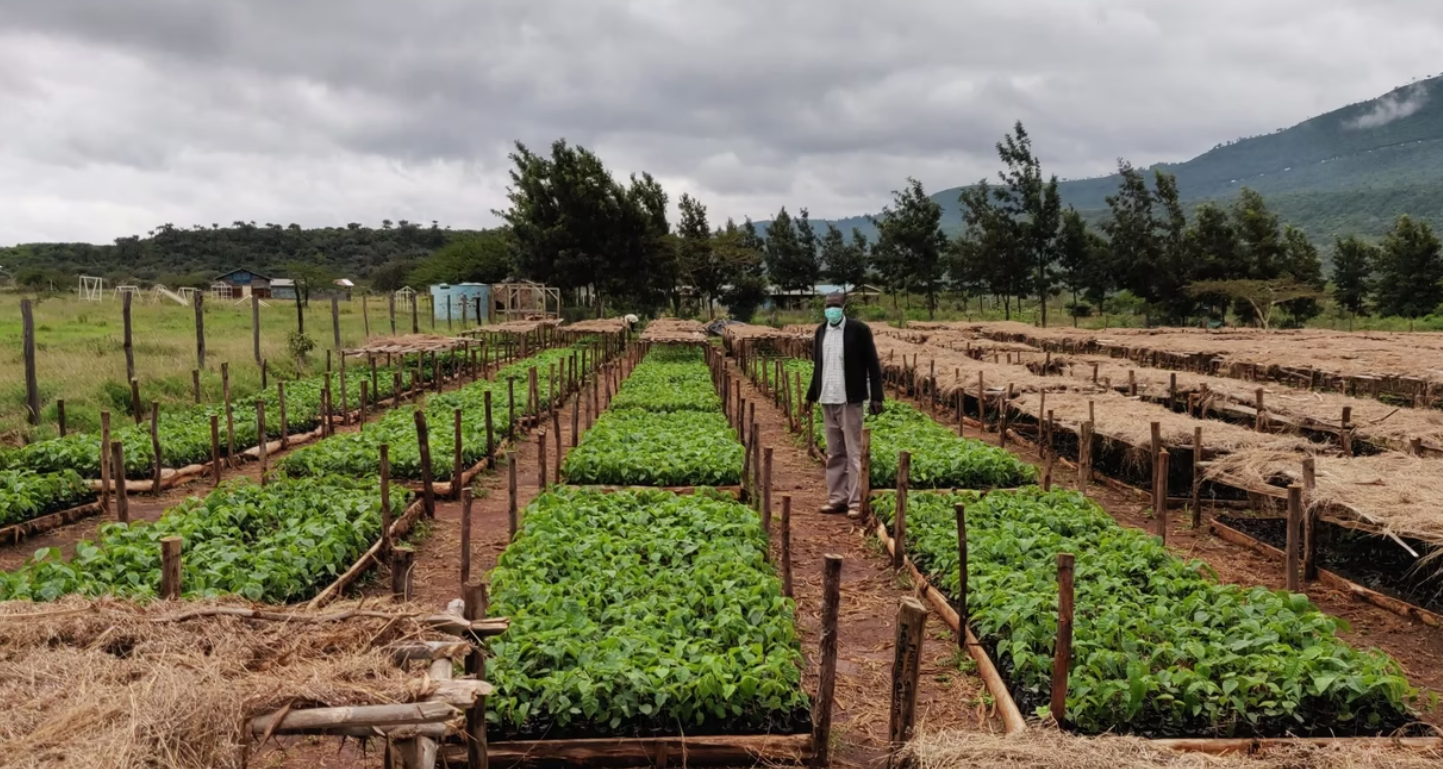
Successful implementation
The Mau forest region of Kenya has suffered decades of deforestation, driven by increasing agriculture, livestock grazing and charcoal production. This has resulted in widespread degradation of land, exacerbating drought and damaging local watersheds.
A 7-year project is ongoing in the region, with the aim of reforesting an area of 5700 hectares. 14.25 million trees will be planted across the site by local community members by 2028.
Efforts are led by Eden Reforestation Projects, a not-for-profit that provides fair wage employment to local populations to become ambassadors of forest restoration. Local people are hired to grow, plant and guard trees to maturity on a massive scale, enabling multiple positive socioeconomic and environment impacts to be unlocked.
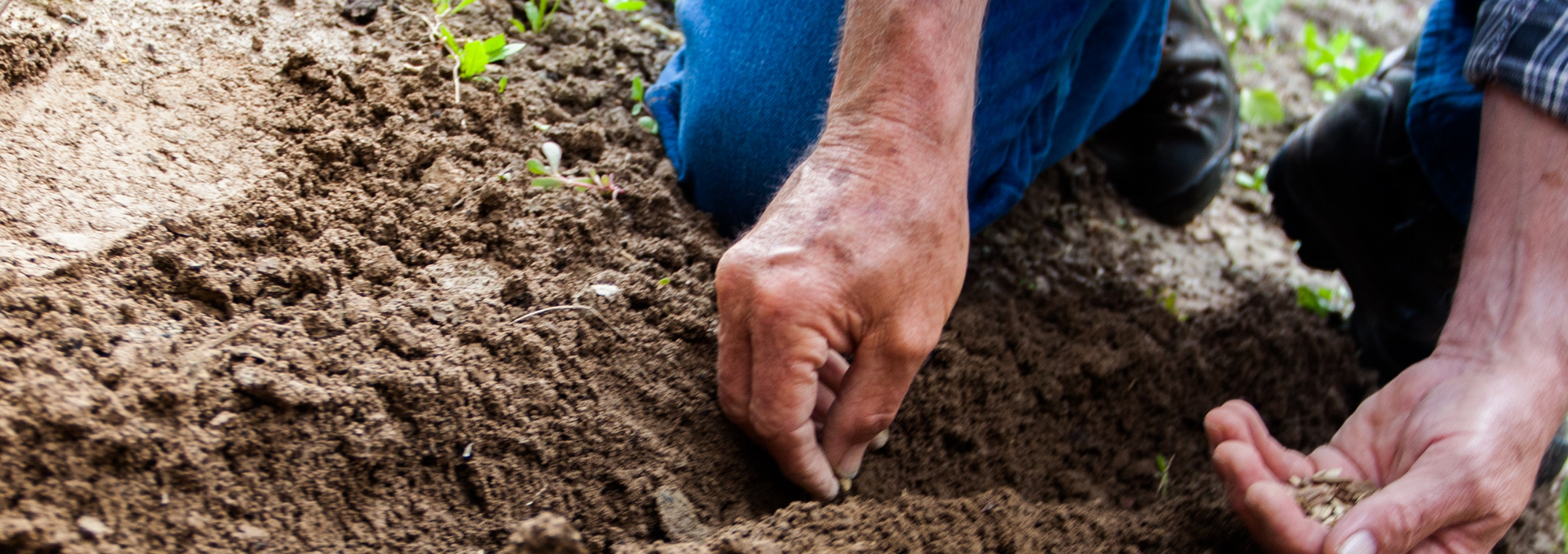
2. Soil carbon sequestration
Overview
Carbon can be removed from the environment by changing land management practices to increase the carbon content of soil.
As illustrated in the diagram below, soil plays a huge role in terrestrial carbon storage, holding twice as much as flora and fauna combined.
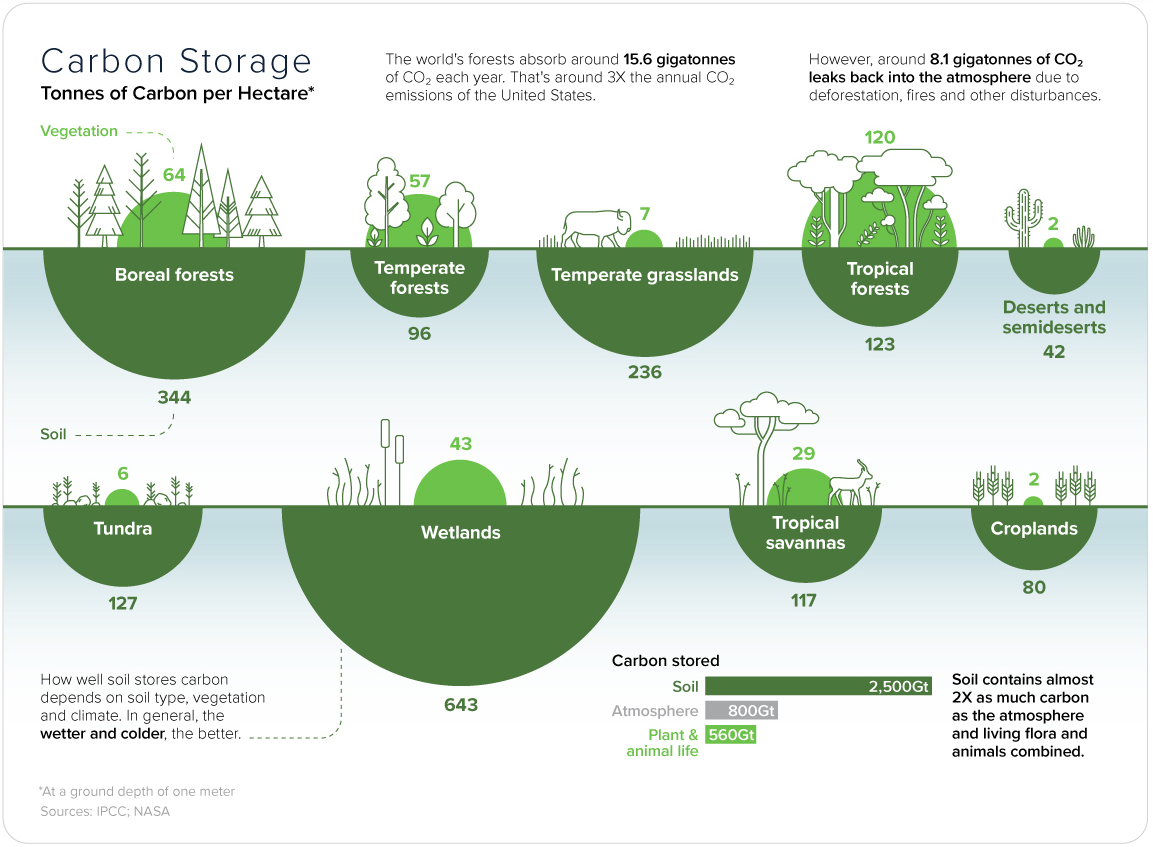
Over the past 12,000 years, land use change has resulted in the loss of some 133 giga tonnes of carbon from our soils. Soil carbon sequestration approaches seek to reverse this trend and rebuild carbon stocks on managed lands.
Many sequestration efforts are focused on agricultural soils, which are depleted in carbon relative to the native ecosystems from which they derived.
There are many agricultural management practices that can promote soil carbon sequestration, including:
- No-till ploughing
- Planting cover crops
- Compost application
- Agroforestry practices (which also improve surface biomass)
The goal of these approaches is to increase and maintain carbon stocks in the form of soil organic matter. As organic matter is prone to decomposition it is essential that long term practices are developed.
A key challenge for soil projects is additionality. As many efforts involve changes to existing practices, it is very difficult to accurately account for the potential carbon removal benefit. Farmers may also be choosing to adopt regenerative approaches as these improve soil quality and water retention, without the need for carbon credits. There is also risk related to indirect carbon leakage, due to pressure placed on other agricultural land in the region.
Sequestering soil organic carbon can however be considered efficient and stable, lasting up to 1000 years and being less vulnerable to ecosystem disturbances like wildfire and disease than forest biomass. This however, requires ongoing development and maintenance of regenerative practices.
There are additionally many co-benefits associated with soil carbon sequestration, including improved water quality, ecosystem restoration, biodiversity preservation, job creation and increased food security. Regenerative agriculture specifically also reduces reliance on fertilizers and allows farmers to better adapt to a changing climate.
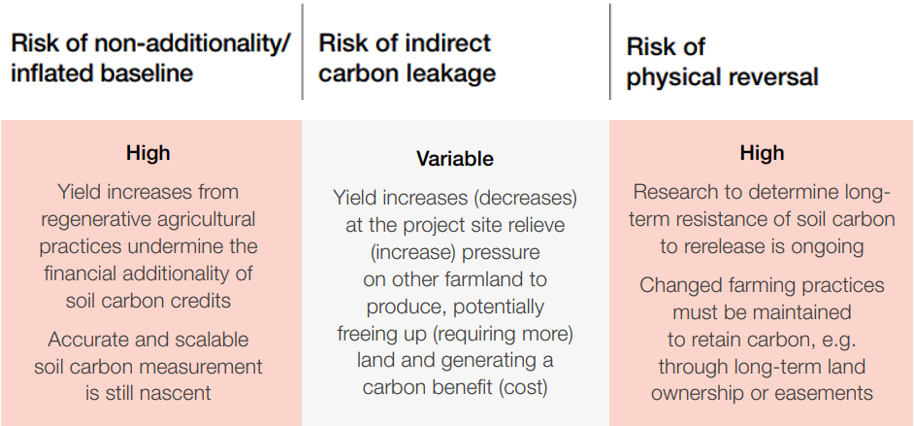
Potential removal capacity and cost
The potential rate of sequestration using current technology is around 3 giga tonnes of CO2 a year globally, at the cost of around $50 a tonne.
Studies estimate that carbon prices between $20 and $100 could drive between 1.5 and 2.6 giga tonnes of this removal potential per year by 2050.
Emerging technologies (such as modified crops with deeper root systems) could even increase soil carbon removal potential to as high as 8 giga tonnes of CO2 a year globally by 2050.
Barriers to accelerating the uptake of soil carbon solutions currently include a lack of:
- Education and support for farmers
- Demonstration projects to learn from
- Supporting policy and financial incentives
- Demonstrated monitoring and verification methods
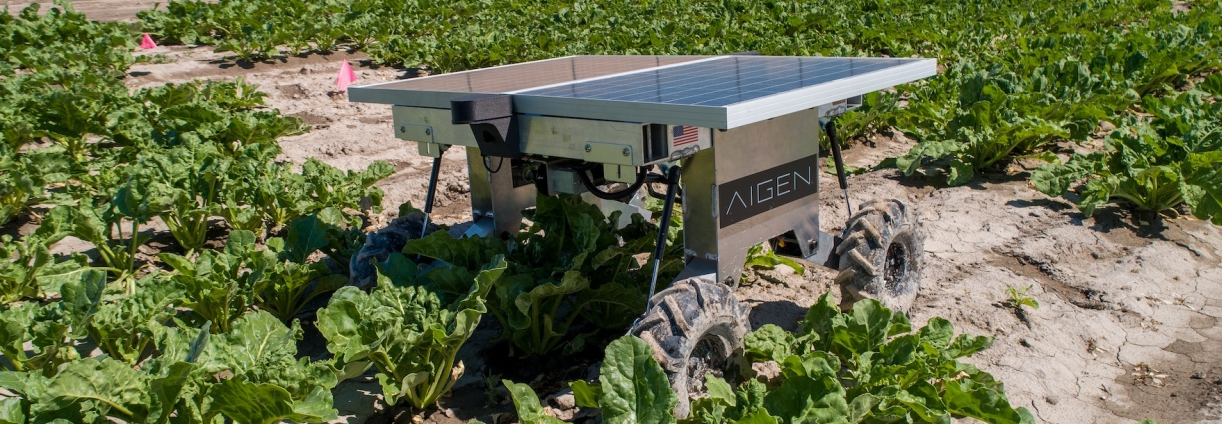
Innovative examples
- Indigo and Farmers Edge have developed large scale carbon farming, assisting the the development of Verra’s soil carbon methodology.
- The Harnessing Plants Initiative is developing crops that can store more carbon in the ground for longer.
- Aigen regenerate soil using solar-powered robots, which either remove or propagate the plants that they encounter, which could assist in more effective land and soil management.
- Ancient Nutrition is entering into a scientific research partnership with Rodale Institute, dedicating 1% of annual revenue towards a 14-year project studying regenerative agriculture.
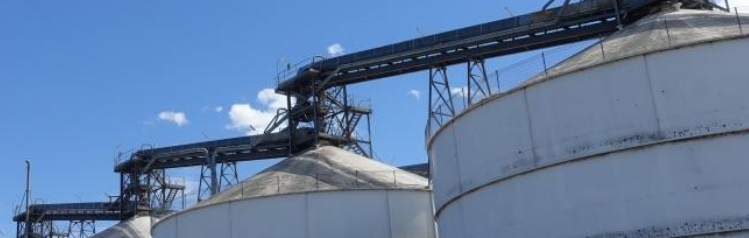
Successful implementation
Mars Petcare has operated a pet food processing facility in Bathurst, Australia, for over 40 years.
As part of their sustainability initiative, they are working with wheat suppliers to pilot approaches to reduce farming emissions while improving soil health.
Project partners plan to recruit 200 farmers over approximately 700,000 hectares to adopt practices including reduced fertilizer use, controlled traffic farming, using cover crops and applying brown manures.
These practices should yield multiple benefits for both farmers and the environment, such as improved soil health, greater resilience to weather shocks, higher yields and reduced CO2 emissions.
By May 2020, it was estimated that Mars will have reduced GHG emissions by 612 tonnes, sequestering 3,20 tonnes of carbon compared to a 2017 baseline.
Full implementation across the 700,000 hectares is calculated to result in 30,000 tonnes of CO2 reduced and 145,000 tonnes of CO2 sequestered.
Research, support and funding is growing for similar farmer-led projects around the world.
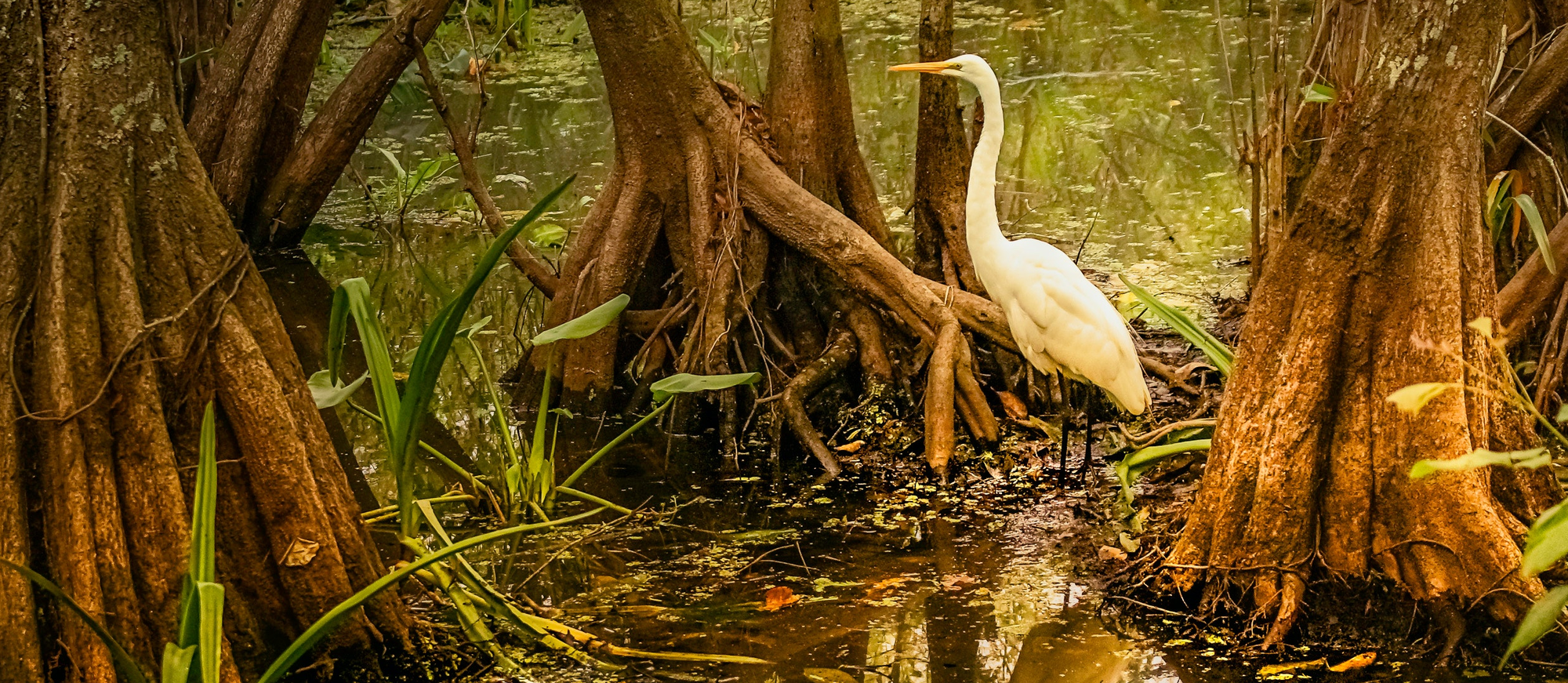
3. Coastal blue carbon
Overview
Coastal blue carbon refers to organic carbon stored in the 49 million hectares of tidally-influenced coastal ecosystems, including salt marshes, mangroves and seagrasses. Carbon sequestration can be increased by managing and restoring these important coastal habitats, illustrated below.
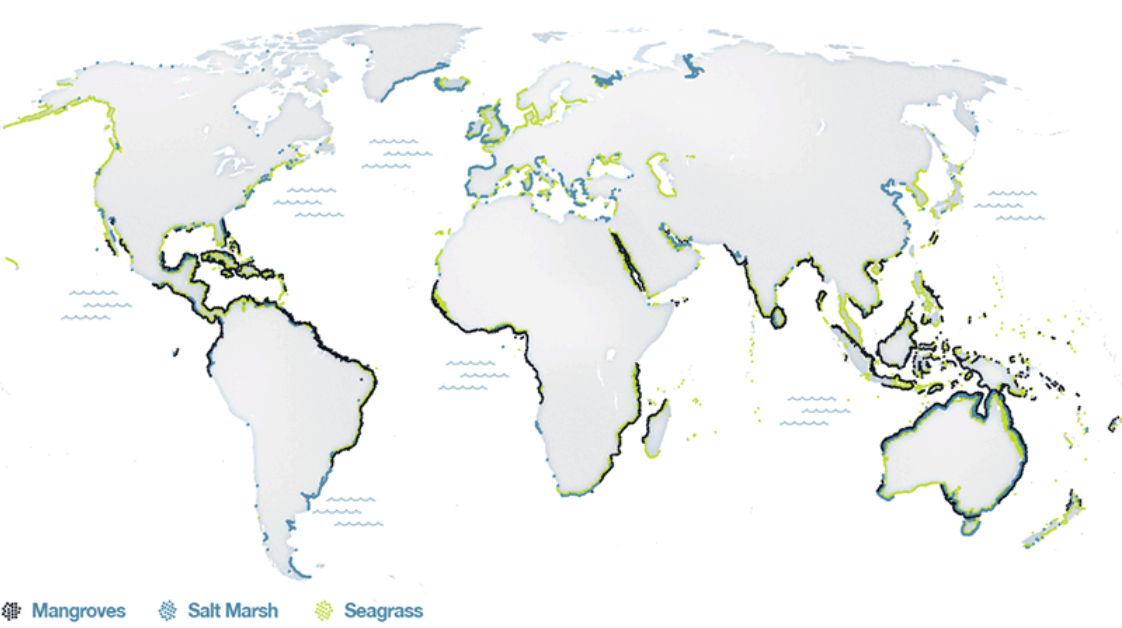
Coastal blue carbon is distinct from other ocean carbon removal methods, which instead seek to enhance the oceans' ability to absorb carbon in ecosystems and ocean chemistry.
Coastal ecosystems hold rich deposits of carbon, primarily in their deep soils. Disturbing one hectare of mangrove is estimated to produce as much emissions as removing three to five hectares of tropical forest. Due to the ongoing drainage and excavation of coastal regions however, these ecosystems have been releasing between 150- 1050 mega-tonnes of CO2 a year back into the atmosphere.
A combination of management activities has the potential to regenerate, preserve and enhance the carbon sequestration of wetlands and to expand the area covered by coastal blue carbon ecosystems. These activities, supported by monitoring and research, can:
- Increase organic carbon density
- Slow erosion of existing wetlands
- Expand wetlands by encouraging coastal flooding
- Manage the effects of sea level rise
Demonstrating the impact of coastal blue carbon projects can be difficult without baseline data and ongoing measurement, reporting and verification (MRV). Much as with soil carbon sequestration, the effect of adjusting management approaches may therefore be difficult to prove.
There is a low risk of blue coastal projects dislocating existing economic activities. However there is a much higher risk associated with long term resilience, as management needs to respond to rising sea levels and ocean temperatures over time.
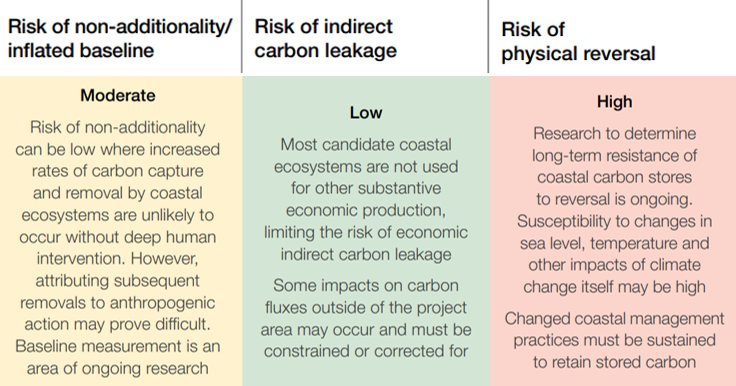
Coastal blue carbon projects may also benefit sustainability development more broadly, such as by providing improved water quality, ecosystem restoration, biodiversity preservation, job creation and climate resilience.
Potential removal capacity and cost
Estimated long-term sequestration rates in coastal wetlands range from 1- 8 tonnes of CO2 per hectare per year.
Globally, coastal wetland and peatland management and restoration could remove between 2.4- 4.5 giga tonnes of carbon a year by 2030.
Cost estimates for coastal blue carbon projects remain highly uncertain, varying widely based on region and technique. Monitoring costs alone could be as much as $100 per tonne of CO2, but reduce to $10- $50 as large scale projects are implemented.
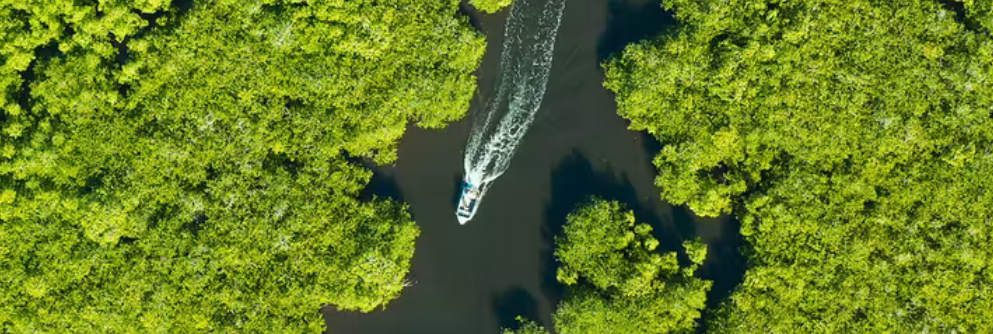
Innovative examples
- Vlinder are currently seeking to develop a pool of tokenized, high-integrity mangrove credits as Mangrove DAO.
- RISCO are assessing and monetizing the value of flood risk reduction and the carbon storage benefits of mangroves alongside Swiss RE and Convergence Insurance companies.
- In Mathurapur, India, carbon credits are providing a new funding mechanism, enabling local communities to drive mangrove reforestation and create a diverse wetland environment.
- Tomago Wetland Restoration project in Australia is measuring the implications of restoring former tidal flood planes for carbon sequestration.
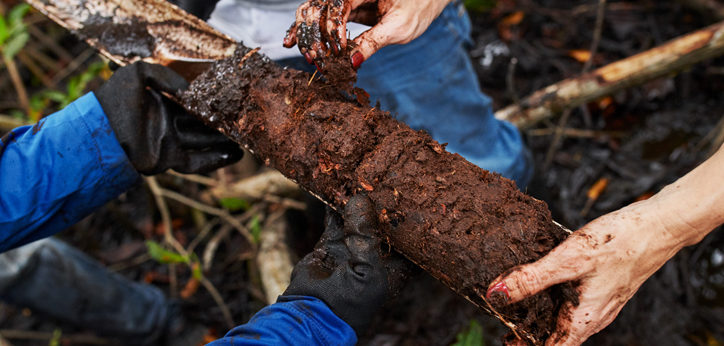
Successful implementation
A mangrove conservation project on Colombia’s Caribbean coast is the first to make use of Verra’s blue carbon credit standard, demonstrating the added value of investing in coastal conservation projects.
The Blue Carbon Project Gulf of Morrosquillo, funded by Apple and run by the non-profit Conservation International, has successfully been managing 7,646 hectares of mangrove forests in the Gulf of Morrosquillo since 2015.
By 2018, the project had been rewarded some 60,000 verified carbon credits, including for carbon stored in the mangroves underwater soils. The project expects to account for just under one million credits over a 30-year period.
Money obtained from the sale of carbon credits will be spent on strengthening ongoing conservation efforts and supporting local community development.
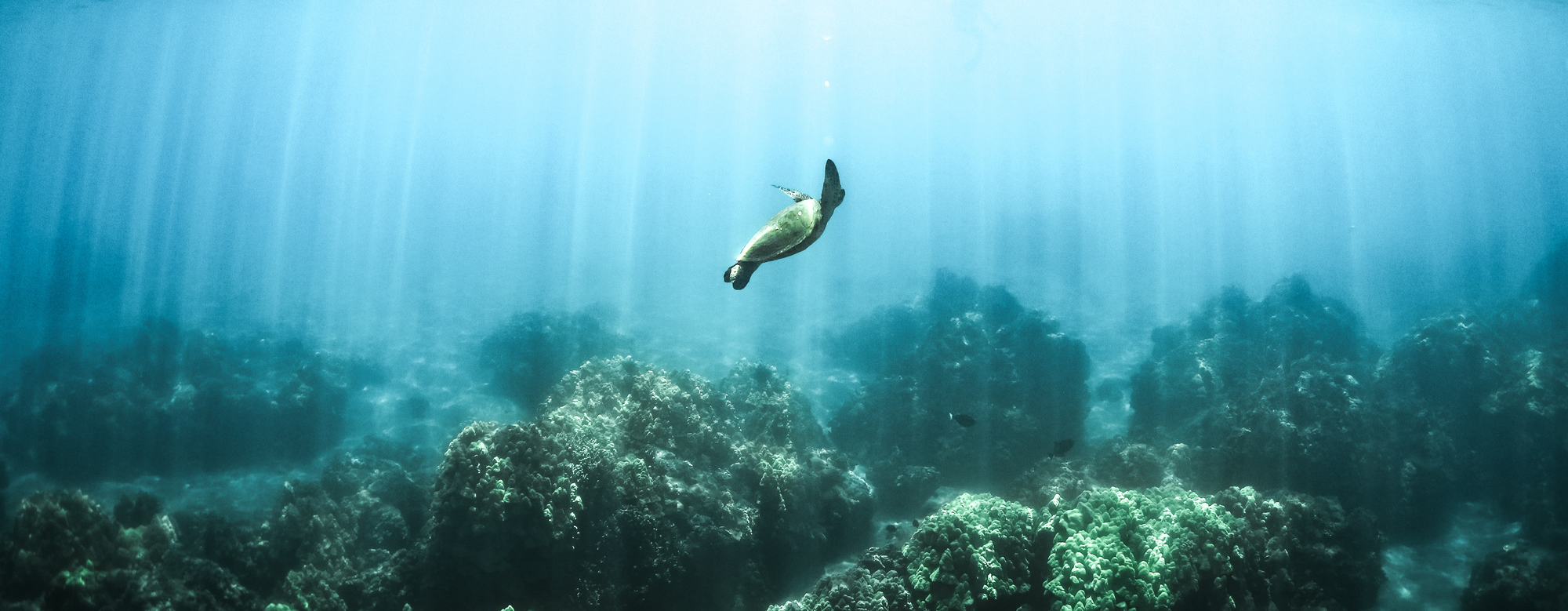
4. Ocean Based Carbon Removal
Overview
Ocean based carbon removal solutions enhance the ocean's physical, geochemical and biological processes to influence CO2 absorption and storage.
Oceans already act as a large natural carbon reservoir, absorbing 25% of annual CO2 emissions. This happens as higher concentrations of CO2 in the air diffuse and dissolve into surface waters, leading to CO2 exchange and potential deep water storage.
Several ocean based solutions are emerging that will accelerate CO2 removal and storage:
- Nutrient fertilization involves the addition of nutrients (iron, phosphorus or nitrogen) to the ocean surface to increase photosynthesis by marine phytoplankton, enhancing CO2 uptake. Once the phytoplankton die, this carbon sinks and is stored in the deep ocean.
- Artificial upwelling and downwelling encourages the exchange of surface water with deep ocean water. This reduces eutrophication and enhances natural carbon uptake and storage.
- Seaweed cultivation crosses over slightly with the section on blue coastal carbon. The restoration and cultivation of seaweed absorbs carbon from surface waters, transferring this to the deep ocean when it sinks and dies.
- Ocean alkalinity enhancement involves chemical alteration of seawater chemistry to increase alkalinity, similar to mineralization approaches applied on land. This increases CO2 removal as it reacts to form solid carbonates.
- Electrochemical approaches pass an electric current through water, encouraging the water to split in the process of electrolysis. This changes the pH of the water to become more alkaline, increasing it’s CO2 absorption potential.
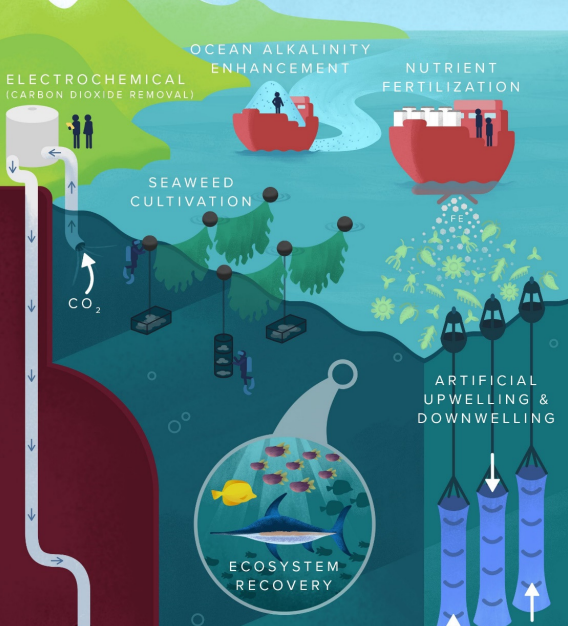
Potential removal capacity and cost
Latest research suggests that the solutions discussed above could collectively account for up to five giga tonnes of CO2 removal a year. These technologies however, remain in the early stages of development, making these predictions very uncertain.
Price also varies between technology, with the lowest cost solution predicted to be nutrient fertilization at around $50 a tonne and the highest electrochemical approaches at $150. As each continues to be developed and deployed, costs are likely to reduce over time.
Ocean based solutions currently face several obstacles to commercial deployment:
- Limited knowledge base around their use
- Issues of social and regulatory acceptability regarding the altering ocean chemistry
- Unknown environmental and social impacts of accelerating ocean CO2 levels
- Lack of adequate monitoring equipment or capacity
More detail on ocean based carbon removal can be found in these excellent resources from NASEM and Climateworks.
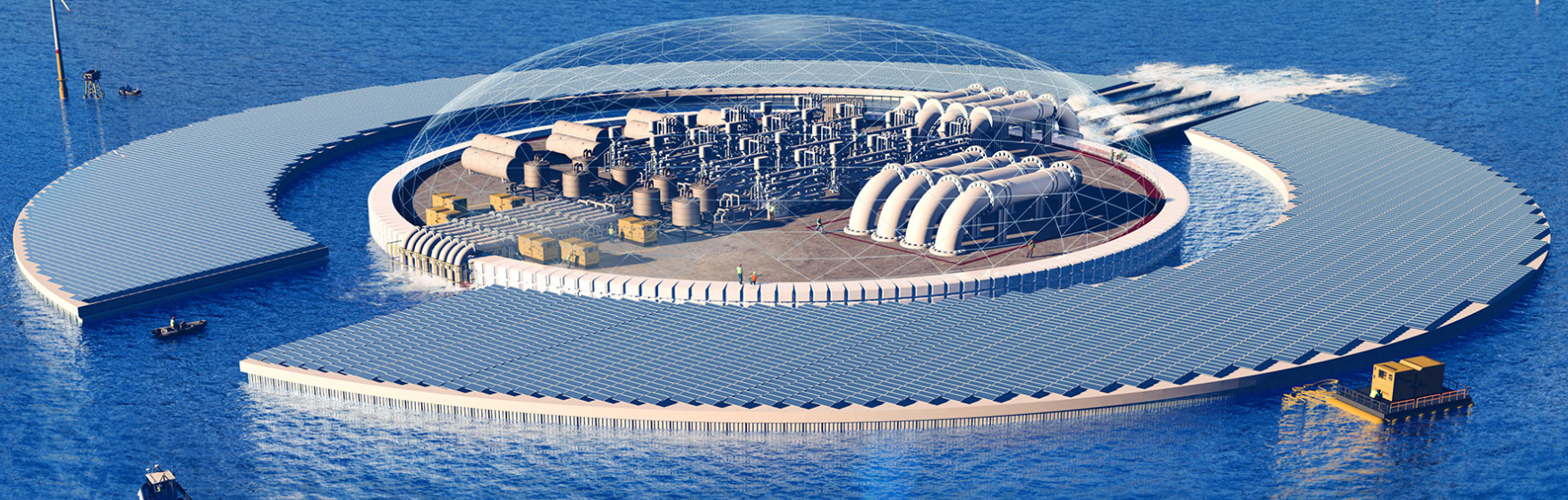
Innovative examples
- Captura are seeking to use solar powered, floating plants to extract CO2 from oceanwater, scalable to billions of tonnes a year.
- Project Vesta are adding carbon removing sand to the ocean, which dissolves to permanently remove CO2 from the atmosphere.
- Running Tide is growing seaweed and then sinking it to the bottom of the ocean, creating a long term store of organic carbon. They recently received offset funding from Stripe and the Chan Zuckerberg Initiative.
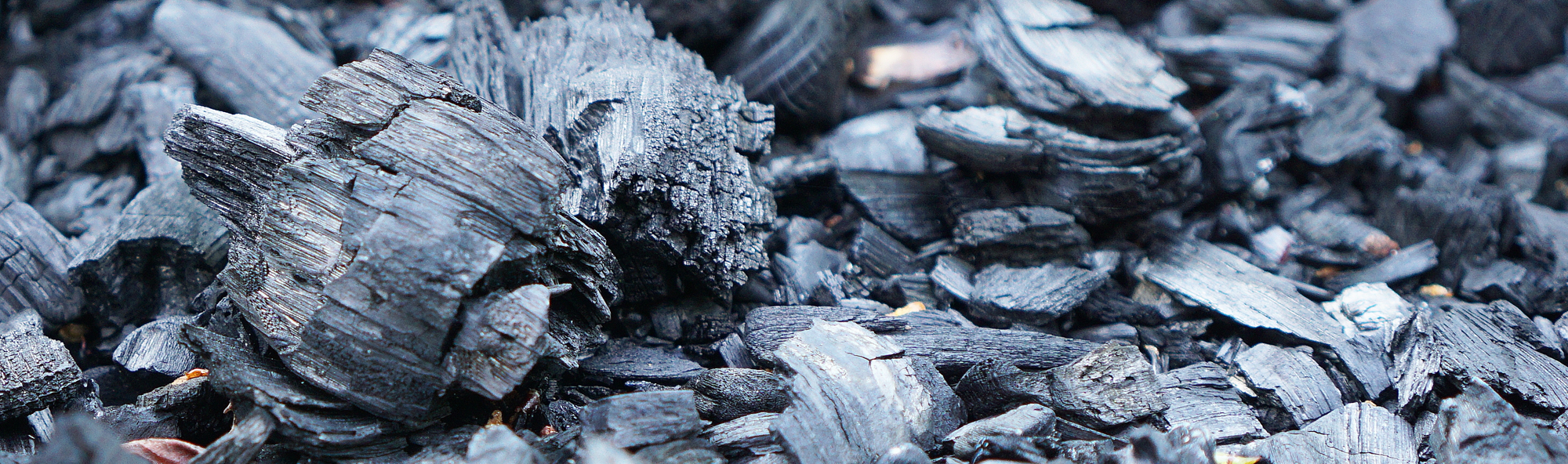
5. Biochar
Overview
Biochar is created by exposing biomass to high temperatures in the absence of oxygen, in a process called pyrolysis. This creates a new substance more durable to biological degradation than biomass, meaning that the organic carbon stored in its structure is returned to the atmosphere more slowly.
Biochar production can be standalone or combined with other processes, such as biofuel production, as depicted below. The biochar produced can then be returned to the soil, either in the system in which the biomass was grown or at another location.
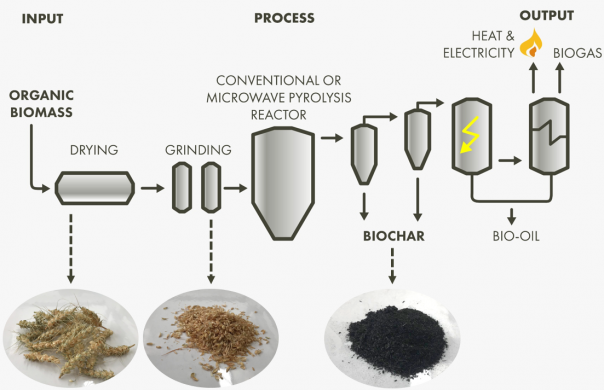
Co-benefits of adding biochar to soil include increased crop yields, which further enhances soil quality and organic carbon content. This enables biochar application to create a positive feedback loop by increasing biomass growth and further increasing carbon removal.
Potential removal capacity and cost
Recent estimates of biochar’s carbon removal capacity range from 1.1 to 3.3 giga tonnes of CO2 a year by 2030. More lifecycle analyses of biochar use is required to better understand this, especially regarding its effectiveness over long time periods.
Commercial scale biochar production remains in the early stages of development. Cost estimates vary significantly and are strongly influenced by the feedstock, application rate, production process, scale and end use of biochar.
Current research indicates a cost between $96 – $1,834 per tonne of biochar, with studies on the CO2 price required for economically viable biochar varying between $30 and $120 a tonne.
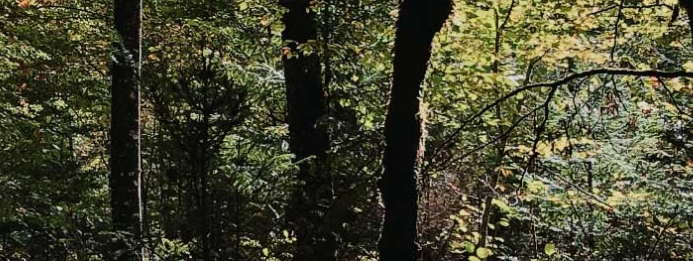
Innovative examples
- The Future Forest Company enhances reforestation projects by applying both biochar and minerals to soil, removing ten times more CO2 than biomass alone.
- Charm uses fast pyrolyzers to change biomass into bio-oil, which is rich in carbon but low in energy content. This oil is then injected underground where it sinks and solidifies for permanent storage.
- Oregon Biochar Solutions manufacture ultra-pure biochar on an industrial scale from logging operations in the Pacific Northwest.
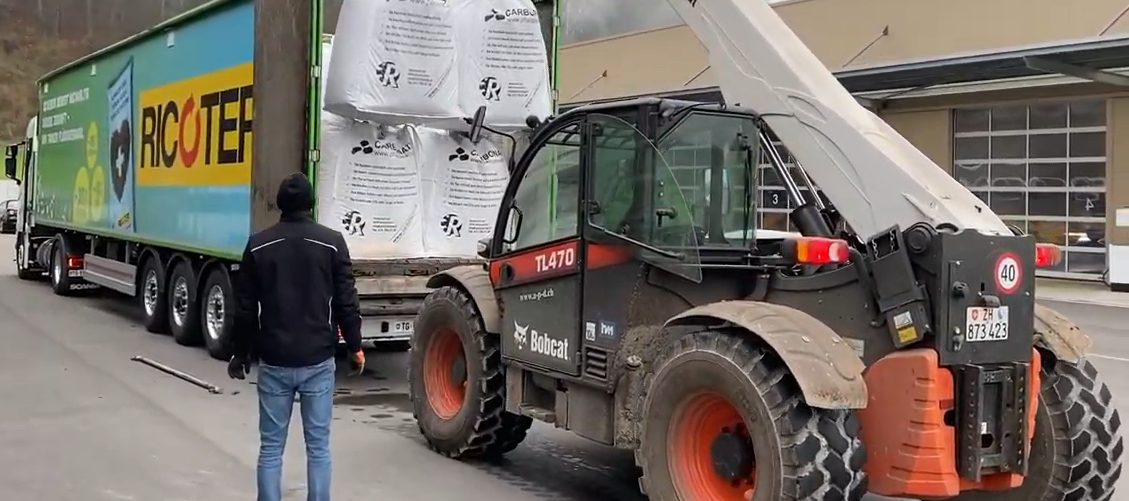
Commercial application
Since June 2019, A-P-D (Auen care service) has been operating a small biochar pyrolysis plant in Zurich, Switzerland.
Toni Meier is a vegetable farmer and owner of the project. He obtains biomass residues from his own farming operations to produce biochar, which he then uses on his own land and sells to farmers in the surrounding area.
Thermal energy generated during the production is also used has part of a newly created district heating network, supplying neighbouring industrial buildings with heat.
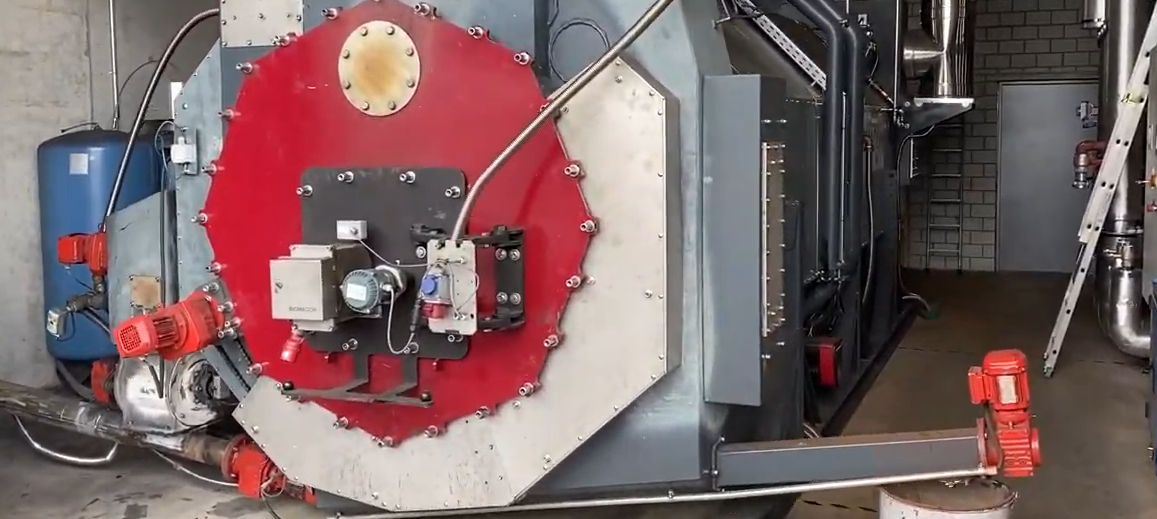
The project is successful in demonstrating the opportunity for biochar to create a revenue stream from waste biomass, improve regional soil quality and create further benefits of sustainable energy generation.
A-P-D is a founding member of AgroCO2ncept, a wider project testing the potential for using biochar on intensively farmed agricultural soils in Switzerland.
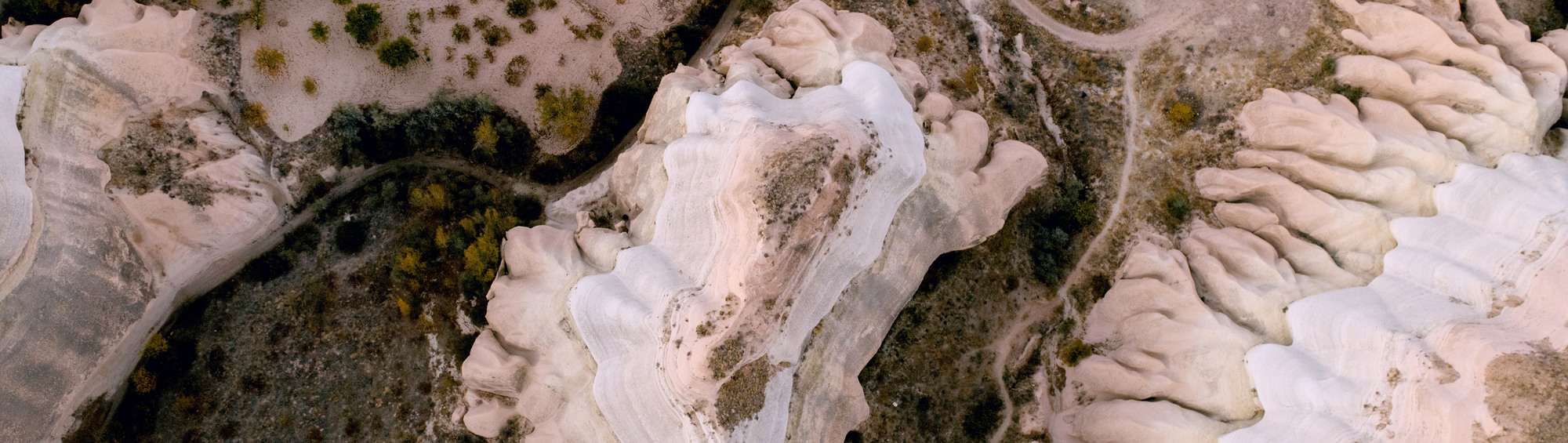
6. CO2 mineralization
Overview
CO2 weathering and mineralization is the processes by which certain minerals react with and form a bond with CO2, removing it from the atmosphere by creating an inert carbonate rock.
Carbon mineralization occurs naturally in alkaline environments. Atmospheric CO2 reacts with rocks that are rich in magnesium and calcium, such as basalt, to form solid carbonates.
Mineralization is fast on a geological timescale and can be replicated in different environments to capture carbon. It presents an appealing pathway for carbon removal as it has a low risk of additionality, a very low risk of carbon leakage and stores carbon very stably.
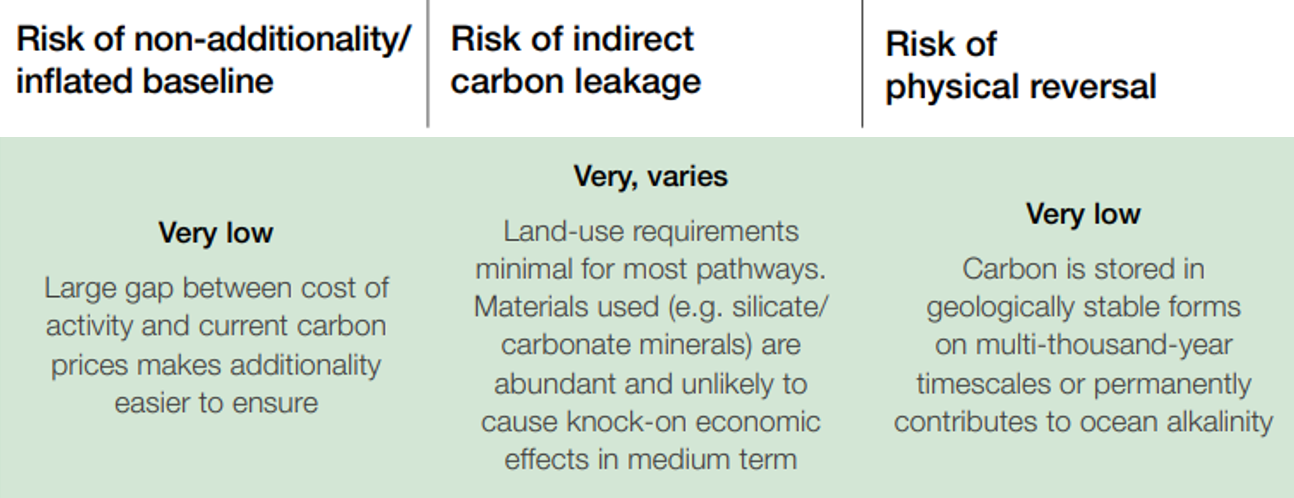
Potential removal capacity and cost
Early estimates speculate that enhanced mineralisation could store 2–4 giga-tonnes of carbon globally per annum, with current annual emissions reaching 60 giga tonnes last year.
There are a broad range of different applications for CO2 mineralisation. These fall into three categories:
- In-situ processes- sending CO2 rich fluids underground to react with alkaline minerals below the surface
- Surficial processes- encouraging enhanced mineralisation by sprinkling alkaline rocks onto land
- Ex situ processes- locking CO2 into industrial wastes or new products, like cement
Given these of different applications, there are many different sequestration opportunities currently at very different price points, as detailed below.
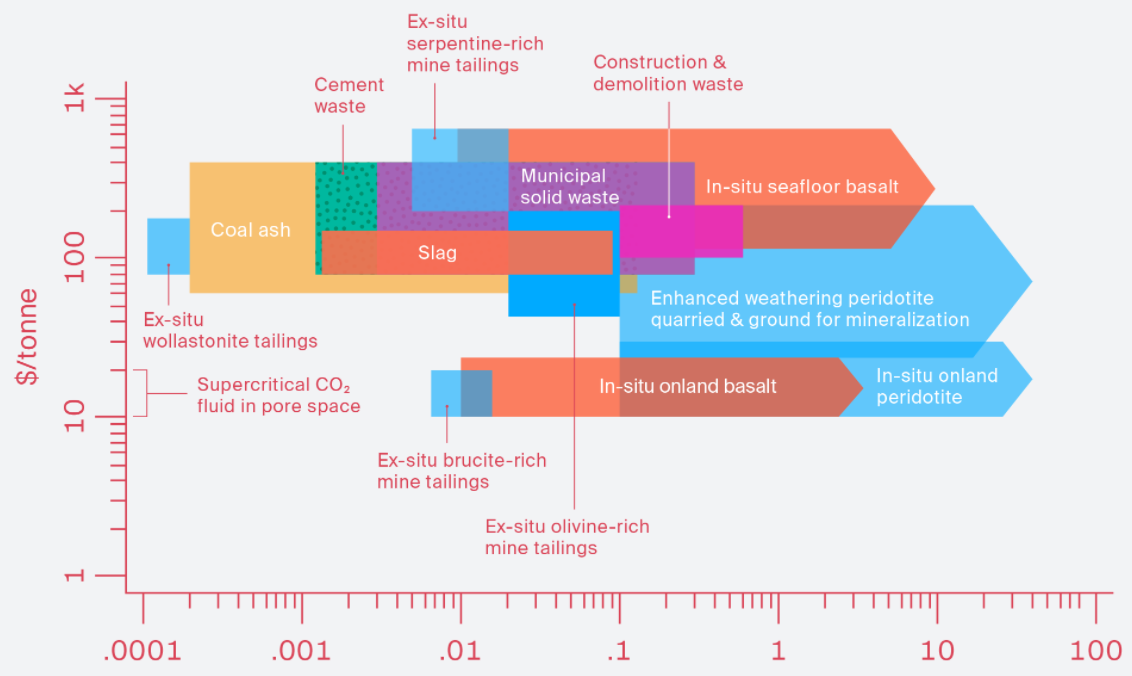
Innovative examples
- To date, Carbfix have successfully stored over 70,000 tons of mineralized CO2 at their injection site in Hellisheiði, Iceland.
- CarbonCure, a Carbon XPRIZE finalist based in Nova Scotia, Canada, injects CO2 into concrete during the concrete curing process.
- Blue Planet Ltd, located in California, dissolves CO2 from flue gas to form aggregate products, ranging from gravel to sand.
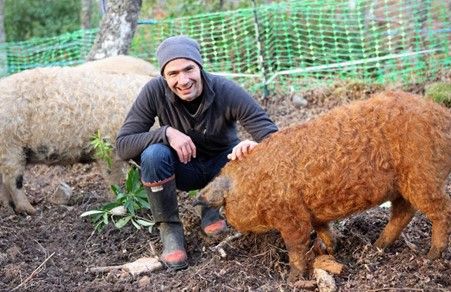
Commercial implementation
The Future Forest Company are pioneering mineral weathering in Scotland. They begin by buying degraded land and restoring it back to health using agroforestry methods, such as rotational livestock grazing.
They then sequester further carbon by spreading basalt fines (small particles of basalt rock) over the forest land.
As rainwater falls, it reacts with the basalt to raise the pH of the soil system. As the pH rises, CO2 dissolved in the rainwater turns into carbonates, that form solid minerals like calcium carbonate.
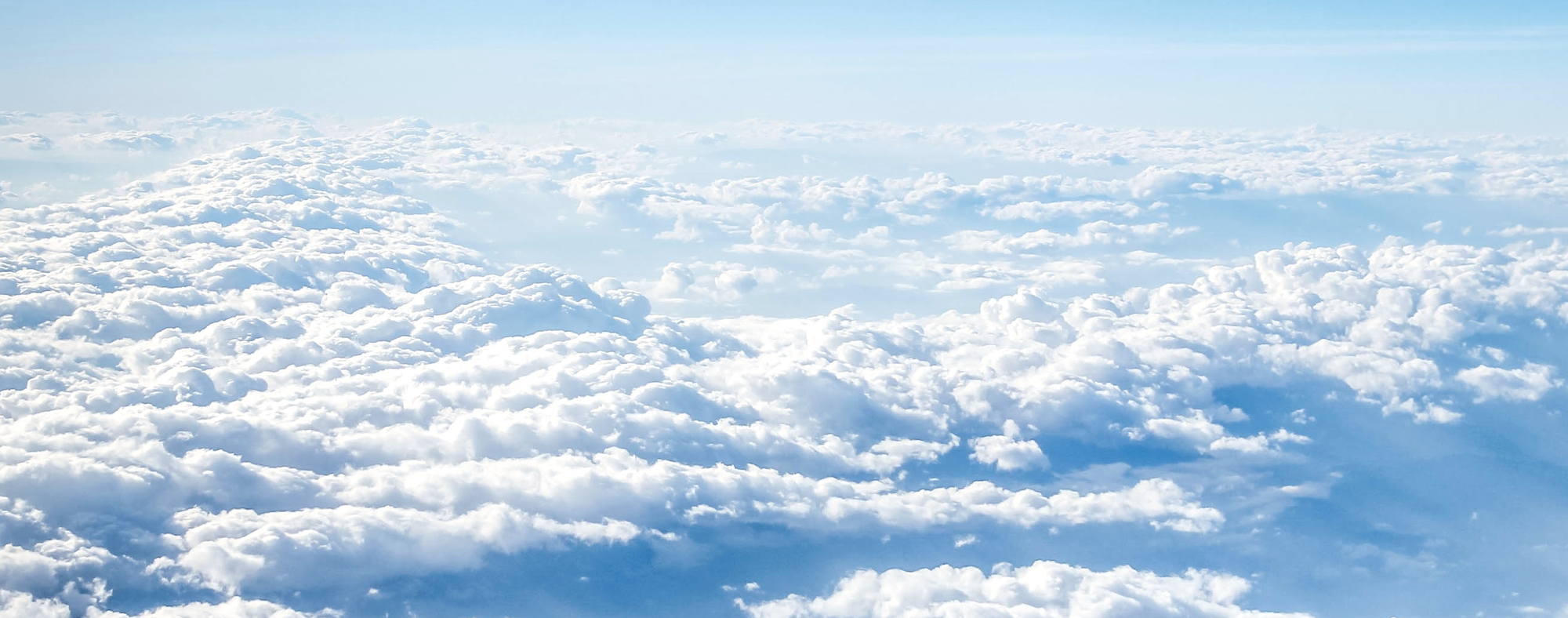
7. Direct Air Capture
Overview
Direct air capture (DAC) refers to technologies that remove CO2 directly from the air. Two main capture agents are used to achieve this:
- Solid sorbents- porous sheets or beads configured to form a bed, working like the catalytic converter in car.
- Water based solvents- liquids that react with CO2 to dissolve it in an aqueous solution.
Fans draw air into contact with these capture agents so that the CO2 collides and binds, while the other components of air move through unimpaired. Air flow is moderated to ensure that the capture agent is able to break the CO2's chemical bonds, forming carbamate or carbonate.
This high-purity CO2 is then recovered from the capture agent using heat, a pressure differential or a chemical displacement reaction. Once the CO2 has been recovered it is then compressed and transported or immediately injected into deep underground storage. This could be as a gas or via a process of mineralization.
DAC approaches to carbon dioxide removal have a low risk of non-additionality given their current cost vs carbon price, with carbon captured unlikely to be physically reversed.
A more moderate risk relates to the energy consumption of DAC technologies needed to power the intake fans and power the process of recovering the CO2 from the capture agent.
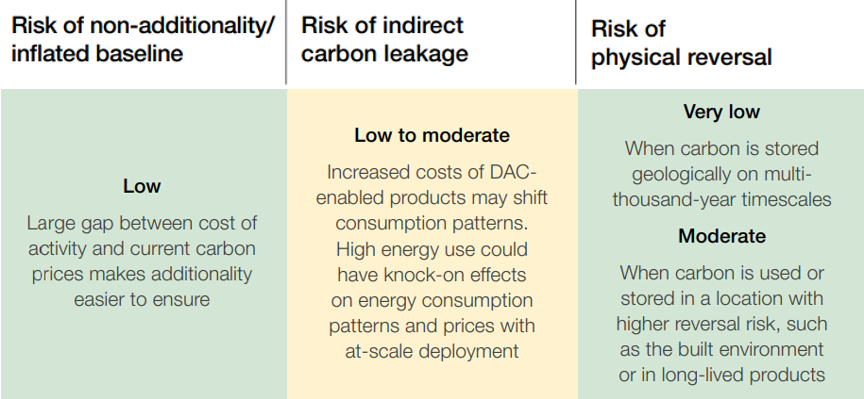
There are sustainability factors that effect large-scale deployment, including:
- High energy use- Depending on the energy source used to recover the captured CO2, relinquishing 1 mega tonne currently requires 180- 500 MW of power a year. The design of a DAC plant therefore may also include a co-located energy which requires additional land and infrastructure.
- Siting issues- DAC needs to be located near to reliable energy infrastructure and the final CO2 storage location if transport costs are to be reduced.
A recent Nature article demonstrated that while some DAC approaches are more sustainable than others, they can take up to five times more resources to develop than switching from gasoline to electric vehicles. A full life-cycle analysis for each DAC project is therefore needed to understand its net carbon benefit.
Potential removal capacity and cost
It is estimated that DACCS removal capacity could vary between 0.5 and 5 giga tonnes globally by 2050.
While DACCS remains expensive at $350- $750 per tonne of CO2 removal, as economies of scale for dominant technologies build, studies estimate that this could reduce to $100 by 2050.
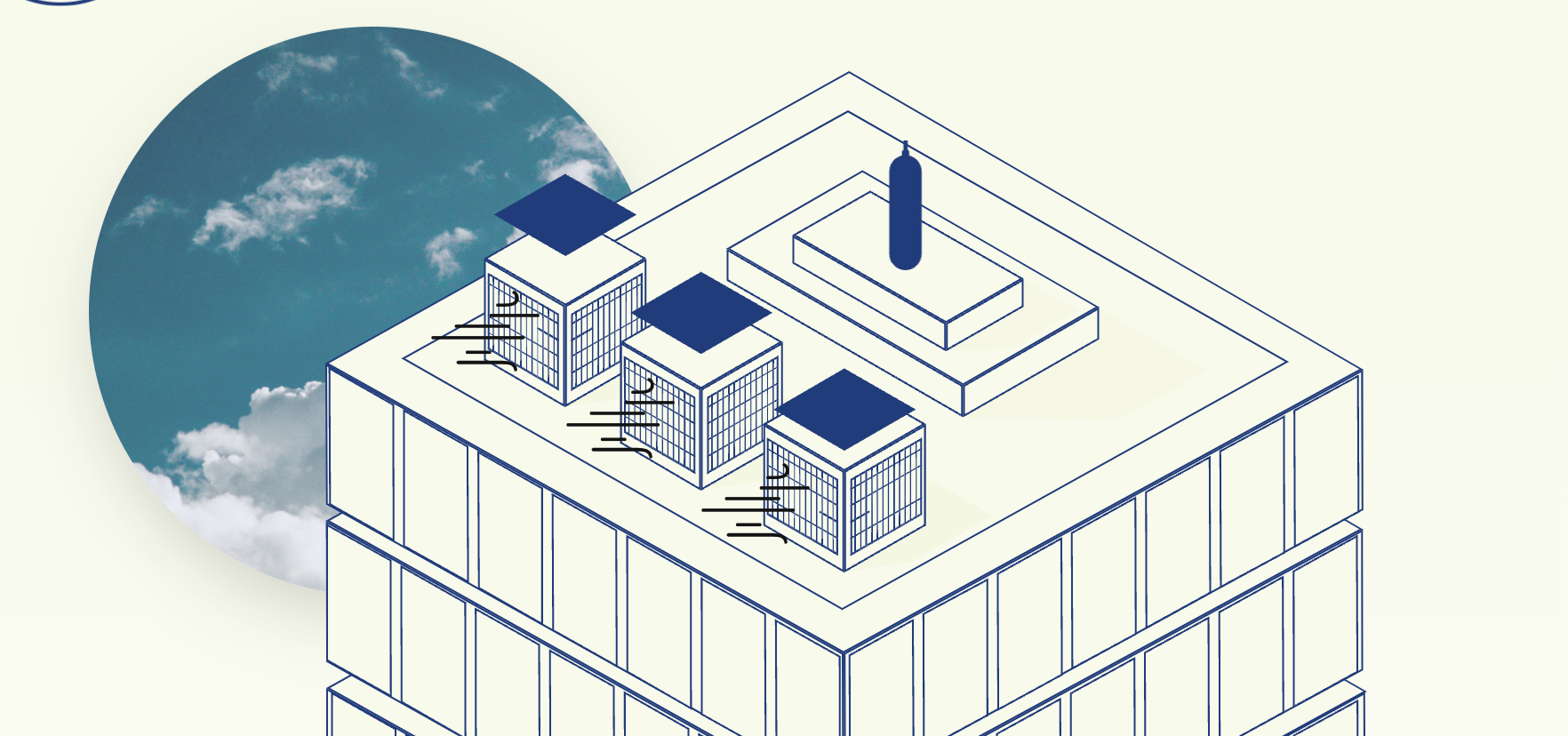
Innovative examples
- Noya retrofit existing cooling towers by adding a water-based solvent that absorbs CO2 from existing processes.
- Heirloom is powered by 100% renewables and reduces energy use by passively exposing air to minerals that capture CO2.
- Solid Carbon aim to deploy DACCS technology installed on an ocean floating platform powered by renewable energy, which will inject captured CO2 into the subsea floor.
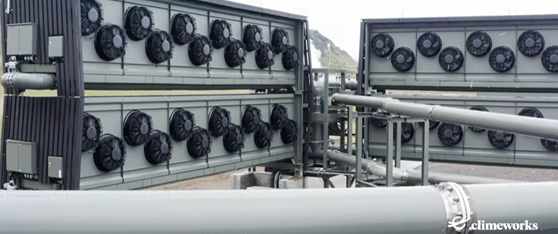
Scaled implementation
Founded in 2010, Climeworks is a Swiss company developing solid sorbent-based DACCS units.
The 2021 Orca project is the world's first large scale demonstration, which mixes extracted CO2 with water and injects it 700 meters below ground. Here it reacts with basalt rock in a process of mineralization developed by project partner Carbfix. This enables carbon to be stored extremely securely, capturing 4000 tonnes of CO2 a year.
Orca uses only low carbon energy and has halved the amount of steel compared to previous demonstration projects. Life cycle analysis of the plant demonstrates that it captures ten times more carbon from the air than it will emit over its lifetime.
LGT (the world’s largest family-owned private banking group) recently signed a ten-year agreement with Climeworks to remove 9000 tons of CO2- the largest ever direct air capture agreement signed by a bank. This was the third ten-year purchase for Climeworks in just 5 months, alongside Swiss RE and Boston Consulting Group.
While the current cost of Climework's removals is still $600 a tonne, they have publicly stated that they anticipate costs to decrease to $200- $300 a tonne by 2024.
They recently received a $650m venture capital injection, which is hoped to help them scale capacity to millions of tonnes a year.
Conclusion
As the role of CDR solutions in delivering net zero becomes more apparent, attention and funding in these solutions will continue to grow. None of these solutions alone provides a silver bullet and represent an interesting mix between nature-based, regenerative practices and technology-led interventions. We look forward to seeing how the bright future of CDR will unfold!
Our next deCarbonized post will dive more deeply into the role of these different removal options, and how, alongside carbon avoidance, they will enable the VCM to most effectively contribute to net zero ✨
Toucan is building the technology to bring the world's supply of carbon credits onto energy-efficient blockchains and turn them into tokens that anyone can use. This paves the way for a more efficient and scalable global carbon market.